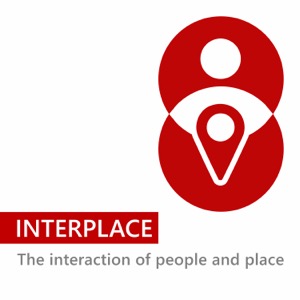
Hello Interactors,
Flying provides a great opportunity to catch up on books and podcasts, but it also brings feelings of guilt. My recent trip likely contributed about 136 hot air balloons' worth of CO2 to the atmosphere. Should I feel guilty, or should the responsibility lie with airlines, manufacturers, and oil companies? We all contribute to global warming, but at least our destination was experiencing an unusually cool July. However, globally, the situation is very different and worsening faster than expected. What’s to be done?
Let’s dig in.
CLIMATE CONUNDRUMS CONFOUND CALCULATIONS
There are two spots on the planet that are not affected by climate change, and I recently flew over one of them. It’s a patch in the ocean just off the coast of Greenland that our plane happened to fly over on a family vacation to Scotland. The other is a small band around the Southern Ocean near Antarctica. I likely won’t be visiting that one.
I learned this on the plane listening to a podcast interview by the physicist Sean Carroll with climate scientist and Director of NASA's Goddard Institute for Space Studies, Gavin Schmidt. Gavin has been at the forefront of climate science, spearheading efforts to quantify Earth's climatic fluctuations, develop sophisticated models for projecting future climate scenarios, and effectively communicate these findings to the public and policymakers.
In this discussion, they talked about the methods currently employed in climate research, while also offering insights into the anticipated climatic shifts and their potential impacts in the coming decades. Gavin is known for bridging gaps between complex science and accessible information. I’m writing this piece to bridge some of my own gaps.
For example, there’s often mention that climate change has created more extreme swings in temperature — that the weather is increasingly varying from extreme heat to extreme cold. In statistics, this is called variance. Some argue this variance may be hard for us to detect because temperatures have been shifting — a phenomenon known as shifting baseline syndrome.
Gavin says there’s more to this question than people realize. He notes that it is relatively straightforward to detect changes in the mean temperature because of the law of large numbers. Temperature varies across three dimensions - latitude, longitude, and altitude. We can calculate an average temperature for any two-dimensional slice of this 3D space, resulting in a single representative value for that area.
This video is a conceptual simulation showing a 3D volume of temperature readings (warmer toward the ground and cool toward the sky). The 2D plane ‘slices’ the cube averaging the values as it encounters them and colors itself accordingly. Source: Author using P5.js with much help from OpenAI.
With enough data, it's clear that there has been a significant warming trend almost everywhere on Earth since the 1970s. Approximately 98% of the planet has experienced detectable warming, with a couple exceptions like the ones I mentioned.
But determining changes in the variance or spread of temperatures is more complex. Calculating variance requires a comprehensive understanding of the entire distribution of data, which requires a larger dataset to achieve statistical confidence. Schmidt points out that while we have enough data to confirm that the distribution of temperatures has shifted (indicating a change in the mean), we do not yet have sufficient data to conclusively state that the variance has increased.
Recent temperature spikes tell this story well. For the last decade or more, many climate scientists have been confident in predicting increased global mean temperatures by looking at past temperatures. The global mean has been predictably increasing within known variances. But in 2023 their confidence was shaken. He said,
“Perhaps we get a little bit complacent. Perhaps we then say, ’Okay, well, you know, we know everything.’ And for the last 10 years or so, [that’s been] on the back of both those long-term trends, which we understand…”
He goes on to explain that they’ve been able to adjust temperature predictions based on past trends and the cyclical variances of El Nino and La Nina. Scientists have boldly claimed,
“’Okay, well, it's gonna be a little bit cooler. It's gonna be a little warmer, but the trends are gonna be up. You know, here's the chance of a new record temperature.’ And for 10 years that worked out nicely until last year. Last year, it was a total bust, total bust like way outside any of the uncertainties that you would add into such a prediction.”
How far outside of known uncertainties? He said,
“…we were way off. And we still don’t know why. And that's a little disquieting.” He added, “…we ended up with records at the end of last year, August, September, October, November, that were, like they were off the charts, but then they were off the charts in how much they were off the charts. So, they were breaking the records where they were breaking the records by a record-breaking amount as well. So that's record breaking squared, if you like, the second order record breaking. And we don't really have a good answer for that yet.”
There is ongoing research into why and some have speculated, but none of them add up.
For example, we’re currently nearing a solar maximum in the sun's 11-year cycle which increases solar irradiance, but that small increase doesn't fully explain the observed changes. Other factors may be at play. For instance, there have been significant shifts in pollution levels in China, and the shipping industry has transitioned to cleaner fuels, which, as hoped, could be influencing climate patterns.
However, Schmidt notes that the quantitative analysis of these factors hasn't yet matched the observed changes. Identifying potential contributors to climate variations is one thing, but precisely quantifying their impacts remains a challenge. Schmidt said climate and planetary scientists hope to convene in December to share and learn more, but the extreme shift remains concerning.
CALCULATING CLIMATE'S COUNTLESS COMPONENTS
The amount of data required to model the climate is daunting. In a separate TED talk, Schmidt reveals that understanding climate change requires considering variables that span 14 orders of magnitude, from the microscopic level (e.g., aerosols) to the planetary scale (e.g., atmospheric circulation). These accordingly have their own orders of magnitude on a time scale, from milliseconds of chemical reactions to weather events over days or weeks to long term changes over millennia, like ice ages or long-term carbon cycles.
Climate models must integrate processes across these scales to accurately simulate climate dynamics. Early models could only handle a few orders of magnitude, but modern models have significantly expanded this range, incorporating more detailed processes and interactions.
Schmidt highlights that climate models reveal emergent properties—patterns that arise from the interactions of smaller-scale processes. For instance, no specific code dictates the formation of cyclones or the wiggles in ocean currents; these phenomena emerge naturally from the model's equations.
But there is a staggering amount of data to model. And it all starts with the sun.
The sun provides 99% of the Earth's energy, primarily in the visible spectrum, with components in the near-infrared and UV. This energy interacts with the atmosphere, which contains water vapor, greenhouse gases, ozone, clouds, and particles that absorb, reflect, or scatter light.
The energy undergoes photolytic reactions. Photolytic reactions are chemical reactions that are initiated or driven by the absorption of light energy which breakdown molecules into smaller units. We couldn’t breathe without it. The earth’s ozone is decomposed into oxygen in the atmosphere through these reactions, which is initiated by sunlight — especially in the stratosphere. This too must be tracked as the Earth rotates, affecting sunlight exposure.
Upon reaching the ground, some sunlight is reflected, by snow for example, or absorbed by oceans and land. This influences temperatures which is then radiated back as infrared energy. This process involves complex interactions with clouds, particles, and greenhouse gases, creating temperature gradients that drive winds and atmospheric motion. These dynamics further affect surface fluxes, water vapor, cloud formation, and associated chemistry, making the entire system highly intricate. And this doesn’t even remotely begin to approach the complexity of it all.
To simplify Schmidt says they capture what they can in a column roughly 25 kilometers high and wide to study the inherent physics. Most of which he says,
“…is just vertical. So, the radiation you can think of as just being a vertical process, to very good order. Convection is also just a vertical process. So, there's a lot of things that you can do in the column that allows you to be quite efficient about how you solve the equations.” Schmidt adds that “each column [can] sit on a different processor, and so you can do lots of things at the same time, and then they interact via the winds and the waves and those kinds of things.”
He said most of the calculations come down to these two sets of equations: Euler and Navier-Stokes. Euler equations are a set of partial differential equations in fluid dynamics that describe the flow of non-viscous and fluids, absent heat exchange. Named after the Swiss mathematician and physicist Leonhard Euler in the 18th century, these simplify the analysis of fluid flow by neglecting viscosity and thermal conductivity, focusing instead on the conservation of mass, momentum, and energy.
Navier-Stokes, named after the 19th century French civil engineer Claude-Louis Navier and the Irish physicist George Gabriel Stokes, is based on Euler’s work but adds viscosity back into the equation. Schmidt says these equations are sometimes used to measure flows closer to the surface of the earth.
This video is a conceptual simulation showing a 3D volume of vectors (randomly changing direction and magnitude) with particles entering the field of vectors. Each particle (e.g. dust, rain, aerosol) gets pushed in the direction of the vector each encounters. You can clearly see the emergent swarming behavior complex adaptive systems, like our atmosphere, can yield. Also present are the apparent challenges that come with measuring and predicting these outcomes. Source: Author using P5.js with much help from OpenAI.
These complex computational models are inherently approximations. They are validated against observations but remain simplifications of reality. This inherent uncertainty is a critical aspect of climate science, emphasizing the need for continuous refinement and validation of models.
And while human-induce climate change denialists like to say the climate models are wrong and not worth considering, Schmidt has a clever retort,
“Models are not right or wrong; they are always wrong, but they are useful.”
NAVIGATING NATURE'S NEW NORMAL
Many wish climate change predictions had the kind of certainty that comes with basic laws of physics. While there are indeed efforts in complexity science to identify such laws, we’re still in the foothills of discovery on a steep climb to certainty.
For example, to even achieve the current level of climate prediction took approximately 30 years of research, involving multiple methods, replication, and more sophisticated physical modeling. This led to accurate calibration techniques for the paleothermometers that measure ice cores which reveal temperatures from around the planet dating back three million years.
While there is some empirical certainty in this — derived from the periodic table, fundamental laws of physics, or observed correlations from spatially dispersed ice core samples — recent extreme variations in global temperatures give reason to question this certainty. These relationships were based on spatial variations observable today, but failed to account for change over time, which behave very differently.
Schmidt says,
“…it turns out that the things that cause things to change in time are not the same things that cause them to change in space. And so empirical relationships that are derived from data that's available rather than the data that you need can indeed lead you astray.”
It begs the question: how far astray are we?
We know over the last one hundred years or so the planet has warmed roughly an average of 1.5 degrees Celsius. This is a number that has been contorted in the media to mean some kind of threshold after which “something” “might” happen. But Schmidt cautions there is no way to know when we hit this number, exactly, and it’s not going to be obvious. Perhaps it already pushed passed this threshold, or it may not for another decade.
He says,
“we are going to continue to warm on the aggregates because we are continuing to put carbon dioxide and other greenhouse gases into the atmosphere. Until we get effectively to net zero, so no more addition of carbon dioxide to the atmosphere, temperatures will continue to climb. The less we put in, the slower that will be. But effectively, our best estimate of when global warming will stop is when we get to net zero.”
Getting to net zero involves significant and radical changes in energy production, industrial processes, and consumption patterns. Moreover, it will require an unprecedented comprehensive and coordinated worldwide effort across all sectors of the economy, institutions, and governments.
This is true even for hypothetical and speculative climate engineering solutions like injecting sulfates into the atmosphere in attempts to cool the planet. According to Schmidt, not only would this require cooperation across borders, so long as we keep spewing emissions into the atmosphere, we’d be forever trying to cool the planet…for eternity or at least until we’ve exhausted all the planet’s fossil fuels.
It’s hard to imagine this happening in my lifetime, if ever. After all, climate change is already disrupting and displacing entire populations and we’re seeing governments, and their citizens, becoming increasingly selfish and isolationist, not collaborative.
As Schmidt admits,
“We're not on the optimum path. We're not on the path that will prevent further damage and prevent the need for further adaptation. So, we're going to have to be building climate resilience, we're going to have to be adapting, we're going to have to be mitigating, and you have to do all three. You can't adapt to an ever-getting-worse situation, it has to at some point stabilize.”
Schmidt says he derives no joy in telling people
“that the next decade is going to be warmer than the last decade and it was warmer than the decade before that.” He says, “It gives me no joy to tell people that, oh yeah, we're going to have another record-breaking year this year, next year, whenever. Because I'm not a sociopath. I'm a scientist, yes, but I'm also a person.”
Schmidt's words resonate deeply, reminding us that behind the data and predictions are real people—scientists, citizens, and future generations—all grappling with the weight of our changing world. As we stand at this critical juncture, we're not just passive observers but active participants in Earth's unfolding story, a story that's leaving its mark on nearly every corner of our planet.
The butterfly effect, as meteorologist Edward Lorenz proposed, isn't just about tornados in Texas being set off by a chain of events from the flap of a butterfly's wings in Brazil; it's a powerful metaphor for our collective impact. Each of us, in our daily choices and actions, creates ripples that extend far beyond our immediate sphere. In a world where only two small patches—one off Greenland's coast and another near Antarctica—remain untouched by climate change, our individual actions carry profound significance.
The path to net zero isn't just about grand gestures or technological breakthroughs. It's about millions of small, intentional actions coalescing into a force powerful enough to alter our trajectory. As we face the challenges ahead, let's remember that our individual agency, when combined, has the potential to create tsunamis of change, even in places we may never visit ourselves.
In the end, it's not just about preserving a habitable planet — it's about preserving our humanity, our connection to each other and to the Earth that sustains us. As we navigate this critical decade and beyond, let's carry with us the knowledge that every action, no matter how small, contributes to the larger narrative of our planet's future. We are all butterflies, and in a world where climate change-free zones are becoming as rare as a family vacation to Antarctica, our wings have never mattered more.
This is a public episode. If you would like to discuss this with other subscribers or get access to bonus episodes, visit interplace.io